SEEd - Physics
Printable Version (pdf)
Course Introduction
Utah Science with Engineering Education Standards
Utah’s Science and Engineering Education (SEEd) standards were written by Utah educators and scientists, using a wide array of resources and expertise. A great deal is known about good science instruction. The writing team used sources including A Framework for K–12 Science Education1, the Next Generation Science Standards2, and related works to craft research-based standards for Utah. These standards were written with students in mind, including developmentally appropriate progressions that foster learning that is simultaneously age-appropriate and enduring. The aim was to address what an educated citizenry should know and understand to embrace the value of scientific thinking and make informed decisions. The SEEd standards are founded on what science is, how science is learned, and the multiple dimensions of scientific work.
Principles of Scientific Literacy
Science is a way of knowing, a process for understanding the natural world. Engineering applies the fields of science, technology, and mathematics to produce solutions to real-world problems. The process of developing scientific knowledge includes ongoing questioning, testing, and refinement of ideas when supported by empirical evidence. Since progress in modern society is tied so closely to this way of knowing, scientific literacy is essential for a society to be engaged in political and economic choices on personal, local, regional, and global scales. As such, the Utah SEEd standards are based on the following essential elements of scientific literacy.
Science is valuable, relevant, and applicable.
Science produces knowledge that is inherently important to our society and culture. Science and engineering support innovation and enhance the lives of individuals and society. Science is supported from and benefited by an equitable and democratic culture. Science is for all people, at all levels of education, and from all backgrounds.
Science is a shared way of knowing and doing.
Science learning experiences should celebrate curiosity, wonder, skepticism, precision, and accuracy. Scientific habits of mind include questioning, communicating, reasoning, analyzing, collaborating, and thinking critically. These values are shared within and across scientific disciplines, and should be embraced by students, teachers, and society at large.
Science is principled and enduring.
Scientific knowledge is constructed from empirical evidence; therefore, it is both changeable and durable. Science is based on observations and inferences, an understanding of scientific laws and theories, use of scientific methods, creativity, and collaboration. The Utah SEEd standards are based on current scientific theories, which are powerful and broad explanations of a wide range of phenomena; they are not simply guesses nor are they unchangeable facts. Science is principled in that it is limited to observable evidence. Science is also enduring in that theories are only accepted when they are robustly supported by multiple lines of peer reviewed evidence. The history of science demonstrates how scientific knowledge can change and progress, and it is rooted in the cultures from which it emerged. Scientists, engineers, and society, are responsible for developing scientific understandings with integrity, supporting claims with existing and new evidence, interpreting competing explanations of phenomena, changing models purposefully, and finding applications that are ethical.
Principles of Science Learning
Just as science is an active endeavor, students best learn science by engaging in it. This includes gathering information through observations, reasoning, and communicating with others. It is not enough for students to read about or watch science from a distance; learners must become active participants in forming their ideas and engaging in scientific practice. The Utah SEEd standards are based on several core philosophical and research-based underpinnings of science learning.
Science learning is personal and engaging.
Research in science education supports the assertion that students at all levels learn most when they are able to construct and reflect upon their ideas, both by themselves and in collaboration with others. Learning is not merely an act of retaining information but creating ideas informed by evidence and linked to previous ideas and experiences. Therefore, the most productive learning settings engage students in authentic experiences with natural phenomena or problems to be solved. Learners develop tools for understanding as they look for patterns, develop explanations, and communicate with others. Science education is most effective when learners invests in their own sense-making and their learning context provides an opportunity to engage with real-world problems.
Science learning is multi-purposed.
Science learning serves many purposes. We learn science because it brings us joy and appreciation but also because it solves problems, expands understanding, and informs society. It allows us to make predictions, improve our world, and mitigate challenges. An understanding of science and how it works is necessary in order to participate in a democratic society. So, not only is science a tool to be used by the future engineer or lab scientist but also by every citizen, every artist, and every other human who shares an appreciation for the world in which we live.
All students are capable of science learning.
Science learning is a right of all individuals and must be accessible to all students in equitable ways. Independent of grade level, geography, gender, economic status, cultural background, or any other demographic descriptor, all K–12 students are capable of science learning and science literacy. Science learning is most equitable when students have agency and can engage in practices of science and sense-making for themselves, under the guidance and mentoring of an effective teacher and within an environment that puts student experience at the center of instruction. Moreover, all students are capable learners of science, and all grades and classes should provide authentic, developmentally appropriate science instruction.
Three Dimensions of Science
Science is composed of multiple types of knowledge and tools. These include the processes of doing science, the structures that help us organize and connect our understandings, and the deep explanatory pieces of knowledge that provide predictive power. These facets of science are represented as “three dimensions” of science learning, and together these help us to make sense of all that science does and represents. These include science and engineering practices, crosscutting concepts, and disciplinary core ideas. Taken together, these represent how we use science to make sense of phenomena, and they are most meaningful when learned in concert with one another. These are described in A Framework for K–12 Science Education, referenced above, and briefly described here:
Science and Engineering Practices (SEPs):
Practices refer to the things that scientists and engineers do and how they actively engage in their work. Scientists do much more than make hypotheses and test them with experiments. They engage in wonder, design, modeling, construction, communication, and collaboration. The practices describe the variety of activities that are necessary to do science, and they also imply how scientific thinking is related to thinking in other subjects, including math, writing, and the arts. For a further understanding of science and engineering practices see Chapter 3 in A Framework for K–12 Science Education.
Crosscutting Concepts (CCCs):
Crosscutting concepts are the organizing structures that provide a framework for assembling pieces of scientific knowledge. They reach across disciplines and demonstrate how specific ideas are united into overarching principles. For example, a mechanical engineer might design some process that transfers energy from a fuel source into a moving part, while a biologist might study how predators and prey are interrelated. Both of these would need to model systems of energy to understand how all of the features interact, even though they are studying different subjects. Understanding crosscutting concepts enables us to make connections among different subjects and to utilize science in diverse settings. Additional information on crosscutting concepts can be found in Chapter 4 of A Framework for K-12 Science Education.
Disciplinary Core Ideas (DCIs):
Core ideas within the SEEd Standards include those most fundamental and explanatory pieces of knowledge in a discipline. They are often what we traditionally associate with science knowledge and specific subject areas within science. These core ideas are organized within physical, life, and earth sciences, but within each area further specific organization is appropriate. All these core ideas are described in chapters 5 through 8 in the K–12 Framework text, and these are employed by the Utah SEEd standards to help clarify the focus of each strand in a grade level or content area.
Even though the science content covered by SEPs, CCCs, and DCIs is substantial, the Utah SEEd standards are not meant to address every scientific concept. Instead, these standards were written to address and engage in an appropriate depth of knowledge, including perspectives into how that knowledge is obtained and where it fits in broader contexts, for students to continue to use and expand their understandings over a lifetime.
Articulation of SEPs, CCCs, and DCIs
Science and Engineering Practices |
Crosscutting Concepts |
Disciplinary Core Ideas |
Asking questions or defining problems:
Students engage in asking testable questions and defining problems to pursue understandings of phenomena.
Developing and using models:
Students develop physical, conceptual, and other models to represent relationships, explain mechanisms, and predict outcomes.
Planning and carrying out investigations:
Students plan and conduct scientific investigations in order to test, revise, or develop explanations.
Analyzing and interpreting data:
Students analyze various types of data in order to create valid interpretations or to assess claims/conclusions.
Using mathematics and computational thinking:
Students use fundamental tools in science to compute relationships and interpret results.
Constructing explanations and designing solutions:
Students construct explanations about the world and design solutions to problems using observations that are consistent with current evidence and scientific principles.
Engaging in argument from evidence:
Students support their best explanations with lines of reasoning using evidence to defend their claims.
Obtaining, evaluating, and communicating information:
Students obtain, evaluate, and derive meaning from scientific information or presented evidence using appropriate scientific language. They communicate their findings clearly and persuasively in a variety of ways including written text, graphs, diagrams, charts, tables, or orally. |
Patterns:
Students observe patterns to organize and classify factors that influence relationships
Cause and effect:
Students investigate and explain causal relationships in order to make tests and predictions.
Scale, proportion, and quantity:
Students compare the scale, proportions, and quantities of measurements within and between various systems.
Systems and system models:
Students use models to explain the parameters and relationships that describe complex systems.
Energy and matter:
Students describe cycling of matter and flow of energy through systems, including transfer, transformation, and conservation of energy and matter.
Structure and function:
Students relate the shape and structure of an object or living thing to its properties and functions.
Stability and change:
Students evaluate how and why a natural or constructed system can change or remain stable over time. |
Physical Sciences:
(PS1) Matter and Its Interactions
(PS2) Motion and Stability: Forces and Interactions
(PS3) Energy
(PS4) Waves
Life Sciences:
(LS1) Molecules to Organisms
(LS2) Ecosystems
(LS3) Heredity
(LS4) Biological Evolution
Earth and Space Sciences:
(ESS1) Earth’s Place in the Universe
(ESS2) Earth’s Systems
(ESS3) Earth and Human Activity
Engineering Design:
(ETS1.A) Defining and Delimiting an Engineering Problem
(ETS1.B) Developing Possible Solutions
(ETS1.C) Optimizing the Design Solution |
Organization of Standards
The Utah SEEd standards are organized into strands which represent significant areas of learning within grade level progressions and content areas. Each strand introduction is an orientation for the teacher in order to provide an overall view of the concepts needed for foundational understanding. These include descriptions of how the standards tie together thematically and which DCIs are used to unite that theme. Within each strand are standards. A standard is an articulation of how a learner may demonstrate their proficiency, incorporating not only the disciplinary core idea but also a crosscutting concept and a science and engineering practice. While a standard represents an essential element of what is expected, it does not dictate curriculum—it only represents a proficiency level for that grade. While some standards within a strand may be more comprehensive than others, all standards are essential for a comprehensive understanding of a strand’s purpose.
The standards of any given grade or course are not independent. SEEd standards are written with developmental levels and learning progressions in mind so that many topics are built upon from one grade to another. In addition, SEPs and CCCs are especially well paralleled with other disciplines, including English language arts, fine arts, mathematics, and social sciences. Therefore, SEEd standards should be considered to exist not as an island unto themselves, but as a part of an integrated, comprehensive, and holistic educational experience.
Each standard is framed upon the three dimensions of science to represent a cohesive, multi-faceted science learning outcome.
- Within each SEEd Standard Science and Engineering Practices are bolded.
- Crosscutting Concepts are underlined.
- Disciplinary Core Ideas are added to the standard in normal font with the relevant DCIs codes from the K–12 Framework (indicated in parentheses after each standard) to provide further clarity.
- Standards with specific engineering expectations are italicized.
- Many standards contain additional emphasis and example statements that clarify the learning goals for students.
- Emphasis statements highlight a required and necessary part of the student learning to satisfy that standard.
- Example statements help to clarify the meaning of the standard and are not required for instruction.
An example of a SEEd standard:
- Standard K.2.4 Design and communicate a solution to address the effects that living things (plants and animals, including humans) experience while trying to survive in their surroundings. Define the problem by asking questions and gathering information, convey designs through sketches, drawings, or physical models, and compare designs. Emphasize students working from a plant, animal, or human perspective. Examples could include a plant growing to get more sunlight, a beaver building a dam, or humans caring for the Earth by reusing and recycling natural resources. (ESS3.C, ETS1.A, ETS1.B, ETS1.C)
Each part of the above SEEd standard is identified in the following diagram:
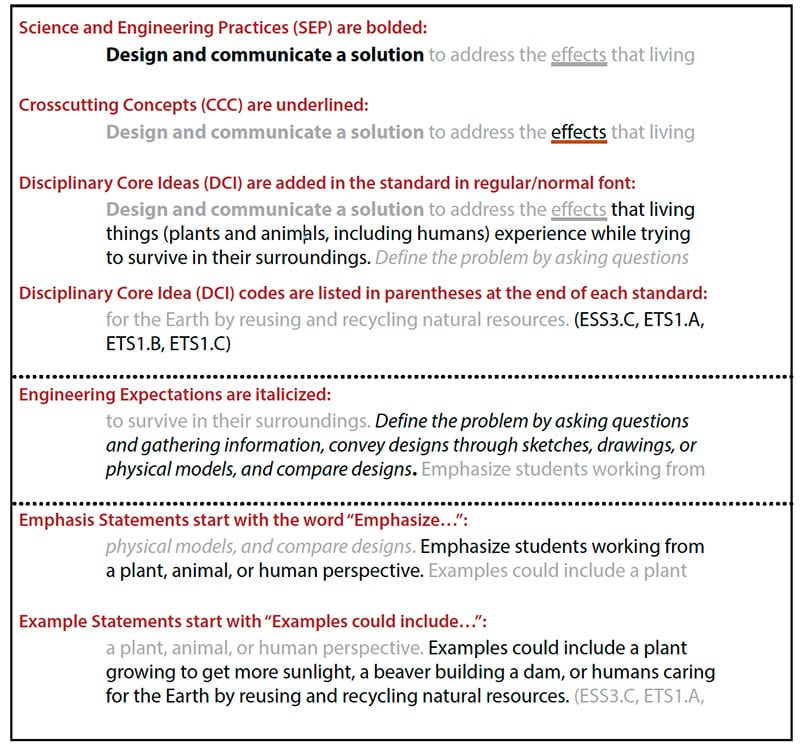
Goal of the SEEd Standards
The Utah SEEd Standards is a research-grounded document aimed at providing accurate and appropriate guidance for educators and stakeholders. But above all else, the goal of this document is to provide students with the education they deserve, honoring their abilities, their potential, and their right to utilize scientific thought and skills for themselves and the world that they will build.
1 National Research Council. 2012. A Framework for K–12 Science Education: Practices, Crosscutting Concepts, and Core Ideas. Washington, DC: The National Academies Press. https://doi.org/10.17226/13165. This consensus research document and its chapters are referred to throughout this document as a research basis for much of Utah’s SEEd standards.
2 Most Utah SEEd Standards are based on the Next Generation Science Standards (NGSS Lead States. 2013. Next Generation Science Standards: For States, By States. Washington, DC: The National Academies Press) http://www. nextgenscience.org
Introduction
The physics SEEd standards explore the foundational principles of physics including forces, energy, fields, and waves. Students analyze and interpret data to determine the cause and effect relationship between the net force of an object and its change in motion. Students develop and use models to illustrate that energy at all levels can be accounted for as a combination of energies associated with motion and relative positions
of objects. Students use mathematics and computational thinking to support the claim that the total momentum of a system is conserved when there is no net force acting on a system. Students plan and conduct investigations to provide evidence that an electric current causes a magnetic field and that a changing magnetic field causes an electric current. Students also engage in argument to support the assertion that electromagnetic radiation can be described either by a wave or a particle model. Additionally, students design and evaluate solutions to problems that exist in these areas.
Core Standards of the Course
Strand PHYS.1: FORCES AND INTERACTIONS
Uniform motion of an object is natural. Changes in motion are caused by a nonzero sum of forces. A "net force" causes an acceleration as predicted by Newton's 2nd Law. Qualitative and quantitative analysis of position, velocity, and acceleration provide evidence of the effects of forces. Momentum is defined for a particular frame of reference; it is the product of the mass and the velocity of the object. In any system, total momentum is always conserved. If a system interacts with objects outside itself, the total momentum of the system can change; however, any such change is balanced by changes in the momentum of objects outside the system. The time over which these paired forces are exerted determines the impact force.
Standard PHYS.1.1
Analyze and interpret data to determine the cause and effect relationship between the net force on an object and its change in motion as summarized by Newton's Second Law of Motion. Emphasize one-dimensional motion and macroscopic objects moving at non-relativistic speeds. Examples could include objects subject to a net unbalanced force, such as a falling object, an object sliding down a ramp, or a moving object being pulled by a constant force. (PS2.A)
Standard PHYS.1.2
Use mathematics and computational thinking to support the claim that the total momentum of a system is conserved when there is no net force acting on the system. Emphasize the quantitative conservation of momentum in interactions and the qualitative meaning of this principle. Examples could include one-dimensional elastic or inelastic collisions between objects within the system. (PS2.A)
Standard PHYS.1.3
Design a solution that has the function of minimizing the impact force on an object during a collision. Define the problem, identify criteria and constraints, develop possible solutions using models, analyze data to make improvements from iteratively testing solutions, and optimize a solution. Emphasize problems that require application of Newton's Second Law of Motion or conservation of momentum. (PS2.A, ETS1.A, ETS1.B, ETS1.C)
Strand PHYS.2: ENERGY
Energy describes the motion and interactions of matter and radiation within a system. Energy is a quantifiable property that is conserved in isolated systems and in the universe as a whole. At the macroscopic scale, energy manifests itself in multiple ways such as in motion, sound, light, and thermal energy. Uncontrolled systems always evolve toward more stable states- that is, toward more uniform energy distribution. Examining the world through an energy lens allows us to model and predict complex interactions of multiple objects within a system and address societal needs.
Standard PHYS.2.1
Analyze and interpret data to track and calculate the transfer of energy within a system. Emphasize the identification of the components of the system, along with their initial and final energies, and mathematical descriptions to depict energy transfer in the system. Examples of energy transfer could include the transfer of energy during a collision or heat transfer. (PS3.A, PS3.B)
Standard PHYS.2.2
Plan and conduct an investigation to provide evidence that the transfer of thermal energy when two components of different temperature are combined within a closed system results in a more uniform energy distribution among the components in the system. Emphasize that uniform distribution of energy is a natural tendency. Examples could include the measurement of the reduction of temperature of a hot object or the increase in temperature of a cold object. (PS3.B)
Standard PHYS.2.3
Develop and use models on the macroscopic scale to illustrate that energy can be accounted for as a combination of energies associated with the motion of objects and energy associated with the relative positions of objects. Emphasize relationships between components of the model to show that energy is conserved. Examples could include mechanical systems where kinetic energy is transformed to potential energy or vice versa. (PS3.A)
Standard PHYS.2.4
Design a solution by constructing a device that converts one form of energy into another form of energy to solve a complex real-life problem. Define the problem, identify criteria and constraints, develop possible solutions using models, analyze data to make improvements from iteratively testing solutions, and optimize a solution. Examples of energy transformation could include electrical energy to mechanical energy, mechanical energy to electrical energy, or electromagnetic radiation to thermal energy. (PS3.A, PS3.B, ETS1.A, ETS1.B, ETS1.C)
Standard PHYS 2.5
Design a solution to a major global problem that accounts for societal energy needs and wants. Define the problem, identify criteria and constraints, develop possible solutions using models, analyze data to make improvements from iteratively testing solutions, and optimize a solution. Emphasize problems that require the application of conservation of energy principles through energy transfers and transformations. Examples of devices could include one that uses renewable energy resources to perform functions currently performed by nonrenewable fuels or ones that are more energy efficient to conserve energy. (PS3.A, PS3.B, PS3.D, ETS1.A, ETS1.B, ETS1.C)
Strand PHYS.3: FIELDS
Fields describe how forces act through space and how potential energy is stored in systems. These take on different forms of electric, magnetic, or gravitational fields, but similarly provide a mechanism for how matter interacts. When two objects interacting through a field change relative position, the energy stored in the field is changed. These fields are important at a wide variety of scales, ranging from the subatomic to the astronomic.
Standard PHYS.3.1
Use mathematics and computational thinking to compare the scale and proportion of gravitational and electric fields using Newton's Law of Gravitation and Coulomb's Law. Emphasize the comparative strength of these two field forces, the effect of distance between interacting objects on the magnitudes of these forces, and the use of models to understand field forces. (PS2.B)
Standard PHYS.3.2
Plan and conduct an investigation to provide evidence that an electric current causes a magnetic field and that a changing magnetic field causes an electric current. Emphasize the qualitative relationship between electricity and magnetism without necessarily conducting quantitative analysis. Examples could include electromagnets or generators. (PS2.C)
Standard PHYS.3.3
Analyze and interpret data to compare the effect of changes in position of interacting objects on electric and gravitational forces and energy. Emphasize the similarities and differences between charged particles in electric fields and masses in gravitational fields. Examples could include models, simulations, or experiments that produce data or illustrate field lines between objects. (PS3.C)
Standard PHYS.3.4
Develop and use a model to evaluate the effects on a field as characteristics of its source and surrounding space are varied. Emphasize how a field changes with distance from its source. Examples of electric fields could include those resulting from point charges. Examples of magnetic fields could include those resulting from dipole magnets or current-bearing wires. (PS3.C)
PHYS.4: WAVES
Waves transfer energy through oscillations of fields or matter. The wavelength and frequency of a wave are related to one another by the speed of travel of the wave, which depends on the type of wave and the medium through which it passes. Waves produce interference as they overlap but they emerge unaffected by each other. The wave model is useful for explaining many features of electromagnetic radiation, and the particle model explains other features. Electromagnetic radiation can be modeled as a wave of changing electric and magnetic fields or as particles called photons. When light or longer wavelength electromagnetic radiation is absorbed in matter, it is generally converted into thermal energy. Because waves depend upon the properties of fields and the predictable transformation of energy, they can be used to interpret the nature of matter and its energy. Waves are utilized to transmit information both in analog and digital forms.
Standard PHYS.4.1
Analyze and interpret data to derive both qualitative and quantitative relationships based on patterns observed in frequency, wavelength, and speed of waves traveling in various media. Emphasize mathematical relationships and qualitative descriptions. Examples of data could include electromagnetic radiation traveling in a vacuum or glass, sound waves traveling through air or water, or seismic waves traveling through Earth. (PS4.A)
Standard PHYS.4.2
Engage in argument based on evidence that electromagnetic radiation can be described either by a wave model or a particle model, and that for some situations one model better explains interactions within a system than the other. Emphasize how the experimental evidence supports the claim and how models and explanations are modified in light of new evidence. Examples could include resonance, interference, diffraction, or the photoelectric effect. (PS4.A, PS4.B)
Standard PHYS.4.3
Evaluate information about the effects that different frequencies of electromagnetic radiation have when absorbed by biological materials. Emphasize that the energy of electromagnetic radiation is directly proportional to frequency and that the potential damage to living tissue from electromagnetic radiation depends on the energy of the radiation. (PS4.B)
Standard PHYS.4.4
Ask questions and construct an explanation about the stability of digital transmission and storage of information and their impacts on society. Emphasize the stability of digital signals and the discrete nature of information transmission. Examples of stability and instability could include that digital information can be stored in computer memory, is transferred easily, copied and shared rapidly can be easily deleted, has limited fidelity based on sampling rates, or is vulnerable to security breaches and theft. (PS4.A)
Standard PHYS.4.5
Obtain, evaluate, and communicate information about how devices use the principles of electromagnetic radiation and their interactions with matter to transmit and capture information and energy. Emphasize the ways in which devices leverage the waveparticle duality of electromagnetic radiation. Examples could include solar cells, medical imaging devices, or communication technologies. (PS4.A, PS4.B, PS4.C)
http://www.uen.org - in partnership with Utah State Board of Education
(USBE) and Utah System of Higher Education
(USHE). Send questions or comments to USBE
Specialist -
Milo
Maughan
and see the Science - Secondary website. For
general questions about Utah's Core Standards contact the Director
-
Jennifer
Throndsen.
These materials have been produced by and for the teachers of the
State of Utah. Copies of these materials may be freely reproduced
for teacher and classroom use. When distributing these materials,
credit should be given to Utah State Board of Education. These
materials may not be published, in whole or part, or in any other
format, without the written permission of the Utah State Board of
Education, 250 East 500 South, PO Box 144200, Salt Lake City, Utah
84114-4200.